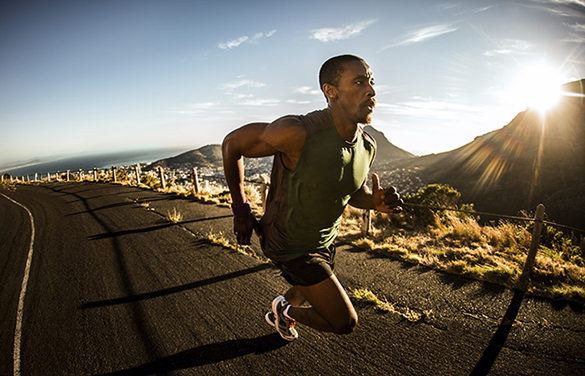
Many athletes aim to become more efficient at burning fat during exercise. This is due to reasons such as preserving glycogen stores, requiring a smaller caloric intake during prolonged activity, and improving body composition.
Another advantage to using fat as a fuel source is that it’s essentially unlimited relative to carbohydrate sources. Generally speaking, lower intensity exercise relies more heavily on fat oxidation for fuel, while higher intensity relies more on carbohydrate. This is due to the rate at which energy in the form of ATP can be created. Fat burning requires the presence of oxygen, while carbohydrate can produce energy with or without oxygen (and produce it more quickly than fats can).
A number of recent studies have shown that performing workouts with low muscle glycogen levels (the body’s carbohydrate “gas tank”) and/or low exogenous carbohydrate availability (reduced carbohydrate intake from things like sports drinks) can result in an increased ability to burn fat during exercise as well as enhancing training adaptations in skeletal muscle. As appealing as that sounds, practical improvements in sports performance are rarely found. Part of this is due to the fact that the low-carb dietary strategies that improve fat-burning also tend to reduce the athlete’s ability to train and perform at high intensities.
If you are familiar with the world of endurance sports, you may have heard the phrase “train low, race high” in reference to training with low carbohydrate availability but racing on a high-carb diet. The latest (and perhaps most promising) strategy for training manipulation is a “train high, sleep low” strategy for carbohydrate intake. Train high, sleep low refers to performing a high-intensity training session after consuming substantial carbohydrate intake throughout the day, but sleeping with low carbohydrate stores by eating a low-carb meal after the high-intensity, glycogen-depleting workout. It has become well–established that the amount of energy available to the muscle before, during, and after exercise can have an effect on fuel metabolism and utilization during exercise as well as on gene expression and cell signaling, and can play an important role in training adaptations. Recent research has begun investigating this unique concept of train-high sleep-low, and has found an upregulation of several markers of fat metabolism.
An important aspect to consider when manipulating carbohydrate intake during training is that it does not need to be an all-or-nothing approach. Rather, one can use an approach to carbohydrate intake that varies each day, in order to ensure that the goals of each training session are met with an appropriate intake. What this means is that high-intensity training sessions that rely quite heavily on carbohydrate for fuel could be performed after consuming adequate dietary carbohydrate, while low-intensity training sessions that are performed to improve fat oxidation can be performed in a carb-depleted state.
With this novel concept of train high, sleep low in mind, investigators set out to see if it could improve endurance performance. This study incorporated three strategies. The first one involved high-intensity training with high-carbohydrate availability aimed at maximizing high-intensity adaptation and performance. The second used overnight carbohydrate restriction to enhance the signaling responses that mediate adaptations after exercise. The third utilized a submaximal training session undertaken in the fasted state in order to promote lipid metabolism.
A novel training and dietary concept called “train high, sleep low” is one nutritional scheme that could support endurance training. It involves coupling high-intensity training with high-carbohydrate availability (training high) followed by overnight carbohydrate restriction (sleeping low). It also includes low-intensity training sessions done after an overnight fast. The goal of this study was to determine if real-world measures of endurance performance in triathletes could be improved using this strategy.
Who and What was Studied?
Twenty-one trained male triathletes (mean VO2max was around 60 ml/kg/min; this is high for recreational athletes but still a level down from professionals) participated in this study, and were divided into a “sleep low” group (n=11) and a control group (n=10). The six-week training protocol (shown in Figure 1) was divided into two three-week phases: three weeks of baseline to assess compliance to the study demands followed by three weeks of training/diet intervention. Dietary intake was the same between groups, with the only difference being the timing of the carbohydrate intake. Food was not provided, but dietary advice was given and intake monitored via food diary.
Figure 1
The training program included six supervised sessions over four consecutive days: high-intensity workouts (8×5 minute cycling intervals at 85% intensity or 6×5 minute running intervals at 10k pace with one minute rest between sets) with high carbohydrate availability in the afternoon and low intensity workouts (60 minutes at 65% intensity) the following morning. The difference between groups was the timing of carbohydrate intake, with the sleep-low group restricting carbohydrate after the evening session and performing the morning workout in the fasted state. In contrast, the control group consumed carbohydrate after the evening workout as well as at breakfast prior to the low intensity morning workout. On the other three days of the week, one low-intensity training session per day was performed for a total weekly training volume of 10-15 hours.
All participants were given dietary instructions, including preset menus. Total daily intake was similar for both groups (about 2700 kcal, six grams of carbohydrate per kilogram of bodyweight and 1.6 grams per kilogram protein per day), but (as shown in Figure 2) intake was allocated differently over the day to achieve high or low carbohydrate availability before and after the various training sessions. During the four consecutive days each week when the intervention was implemented, the sleep-low group did not consume carbohydrate from the afternoon snack before the high intensity workout session until after the completion of the low intensity session. The evening meal for the sleep-low group did not contain any carbohydrate, and the low intensity sessions the next morning were performed after an overnight fast. After the morning workout, participants consumed a large amount of carbohydrate at breakfast, lunch, and as a snack before the next workout. The control group received carbohydrate throughout the recovery period as well as drinking Gatorade sports drink during each training session. Additionally, both groups consumed a high protein drink before going to bed.
Figure 2
To measure the results of this intervention, a number of performance tests were done that attempted to simulate racing and detect meaningful changes in endurance performance. A submaximal test was performed at 70% of maximal aerobic power in order to determine cycling efficiency, which quantifies how much of the energy expended during cycling ends up pushing the pedals rather than just generating heat. A second test was performed to determine supramaximal cycling power by having participants pedal as long as possible at 150% of maximal aerobic power. Finally, a field-based simulation of a triathlon race was performed (but without the swim portion). Participants cycled for 40 minutes at 70% of maximal aerobic power followed immediately by a 10 kilometer simulated running race on an indoor track, during which they were allowed ad libitum consumption of Gatorade.
Trained triathletes were divided into two groups that consumed the same daily carbohydrate intake (six grams per kilogram of bodyweight per day) but with different timing throughout the day. The “sleep low” strategy consisted of a high-intensity interval training session in the evening with high-carbohydrate availability, overnight carbohydrate restriction, and a low intensity aerobic workout in the morning undertaken in the fasted state. The control group followed the same training program but with high carbohydrate availability throughout training sessions. The performance of each group was measured using a battery of endurance tests before and after the intervention.
What Were the Findings?
The main results are shown in Figure 3. The sleep-low group had significantly better performance in the 10 km run after the intervention, while the control group did not have any improvements over their baseline. The average improvement in 10 kilometer running time was 3% (PRE: 40:23 min:s; POST: 39:10 min:s). On the supramaximal test, the sleep-low group improved their time to exhaustion by 12.5% (PRE: 52.7 s; POST: 57.8 s), while no changes were observed in the control group. A decrease in perceived exertion was also observed for the sleep-low group during both the submaximal test and the cycling portion of the simulated triathlon, while no significant changes were seen in the control group. In contrast, the sleep-low group reported higher perceived exertion values during all low-intensity training sessions (which were performed in a fasted state) compared with the control group. During submaximal exercise at 70% intensity, the sleep-low group improved their cycling efficiency and had a decrease in both heart rate and carbohydrate oxidation.
Figure 3
Body composition was measured by DXA, and no changes in fat-free mass were seen in either group. But interestingly, in spite of similar calorie and macronutrient intake, there was a significant decrease in body mass (from 70.6 kilograms to 69.6 kilograms) in the sleep-low group that was due to a loss of fat mass (from 9.70 kilograms to 8.86 kilograms of fat mass). No changes in body mass or fat mass were detected in the control group over the course of training. Additionally, plasma epinephrine (also known as adrenaline) concentrations increased during the training period compared to baseline for the sleep low but not the control group.
The sleep-low group had improvements in submaximal cycling efficiency, supra-maximal cycling to exhaustion, and 10 kilometer running performance, as well as decreased fat mass. No performance changes were seen in the control group.
What Does the Study Really Tell Us?
“Short-term periodization of dietary CHO availability around selected training sessions promoted significant improvements in submaximal cycling economy, as well as supra-maximal cycling capacity and 10 kilometer running time in trained endurance athletes.”
This study offers the first evidence of practical performance improvements from this new method of carbohydrate periodization for trained endurance athletes. Previous strategies of manipulating carbohydrate availability, such as training on a low carb diet and racing on a high carb diet have been intriguing mechanistically, but not so useful for practical performance improvements. This is because extended periods of training with low carbohydrate availability can lead to the down-regulation of carbohydrate metabolism in favor of an increase in fat metabolism. While this may sound good, it does not improve performance capacity. Instead, it impairs rates of muscle glycogenolysis (carb breakdown in the muscle) and limits high-intensity ATP (energy) production. This is because low-intensity aerobic exercise can use fat or carbohydrate for fuel, but high-intensity exercise relies almost exclusively upon the anaerobic metabolism of carbohydrate.
This study demonstrates clear performance improvements after three weeks of manipulating the timing, but not the amount, of dietary carbohydrate. Every participant in the sleep-low group improved their performance in running 10 kilometers (average improvement: 73 seconds), while no improvement was recorded for the control group.
To address the potential problems of down-regulating carbohydrate metabolism in favor of up-regulating fat metabolism, participants in this study performed the high-intensity training sessions with adequate carbohydrate stores and the low-intensity training sessions after a period of carbohydrate restriction. Another key aspect of this study is the fact that the three high-intensity training sessions per week were followed by restricted carbohydrate intake but adequate protein intake. The protein allows muscle repair after the strenuous workout while reduced carbohydrate intake allows beneficial adaptations to occur in the mitochondria. The high-intensity workout will substantially deplete muscle and liver glycogen levels, which will be further reduced after an overnight fast. Performing the low-intensity workout the next morning in the fasted state will maximize the beneficial effects of a low intensity workout on fat oxidation, thus optimizing the benefits from both the high and low-intensity training sessions throughout the week.
Another noteworthy aspect of this study is that improvements in performance were observed in trained endurance athletes, where measurable enhancements are difficult to attain. All participants had been competing in endurance events for longer than two years and were training a minimum of 10 hours per week. A three-week training block would not be expected to result in significant performance improvements, as evidenced by the lack of changes in the control group.
This study was not blinded, so the participants would have been aware that they were taking part in a novel exercise and dietary protocol. It is possible that the changes in perceived exertion were due in part to a placebo effect (higher perceived exertion during the morning training sessions for the group that was fasting, and lower during the performance testing). In addition to the observed performance improvements, the sleep-low group also had a decrease in body mass (about one kilogram after three weeks) that was attributed to a decrease in fat mass. No changes in body weight or body composition occurred in the control group. Even though the participants were self-reporting their food intake, there were no differences in intakes between groups and no reason to think that one group would be under or over-reporting more than the other.
This study offers a novel and practical strategy for trained endurance athletes and demonstrates clear performance improvements after three weeks of manipulating the timing, but not the amount, of dietary carbohydrate.
The Big Picture
This is the first study to show such marked improvements in endurance performance in response to a training regimen that included sessions with low carbohydrate availability. This is likely due to combining high-intensity training with high carbohydrate availability and low-intensity training with low carbohydrate availability, to take advantage of specific physiological responses from the different stimuli. While each of these strategies have been proposed and practiced individually, there had yet to be a study combining them and showing efficacy both in the lab and in the field.
Two studies have reported some type of performance improvements following a “train low” training strategy, however, a number of methodological differences make them difficult to compare to the current study. One study found an increased time to exhaustion in a knee extension exercise after 10 weeks of training twice every second day, with one of the sessions undertaken with depleted glycogen stores. However, these were untrained participants that were focused solely on a single leg training protocol with the other leg serving as the control. Another study looked at two weeks of performing high-intensity interval training sessions two times per day, the second of which was performed with low carbohydrate availability. Sessions were performed three times per week, by active but untrained participants. Improvements in cycling time trial power output were greater in the group that performed the second interval session with reduced carbohydrate availability compared with the control group that was able to refuel with carbohydrate prior to the second training session. In contrast, no differences were seen between groups for improvements in a repeated all-out sprint test (5 x 15 seconds).
Studies in trained populations using train-low protocols have not shown performance improvements beyond what was attained with a more traditional high-carbohydrate approach, despite improved fat oxidation during submaximal intensities. This highlights the fact that too much emphasis placed on one aspect of endurance performance, such as improving fat-burning capacity, can inadvertently have a negative effect on another aspect, like maximal-intensity anaerobic performance. One reason that this new study may have found improvements in performance when other train-low studies have not, is that previous studies performed high-intensity training with low carbohydrate availability, which would reduce the intensity of work that could be completed. In contrast, this current study reduced carb availability only for low-intensity workouts.
During submaximal cycling, the sleep-low group improved their efficiency and had decreased heart rates at a submaximal work output. This suggests a decrease in the relative energy cost of a given exercise intensity, possibly due to changes in mitochondrial enzyme activity. Previous research has shown that decreasing the energy cost of cycling can improve running performance undertaken immediately after cycling. This may explain part of the improvements seen in the 10 kilometer running performance.
Mechanisms of action were not investigated in this study, but a previous study that used a similar strategy has looked at some of the cellular and molecular mechanisms at play. Withholding carbohydrate overnight may have extended the duration of the exercise-stimulated enhancement of specific genes involved in mitochondrial biogenesis (the process by which new mitochondria are created). Undertaking the low-intensity exercise sessions in the fasted state with low muscle glycogen has also been shown to magnify the training response and improve lipid oxidation by enhancing key signaling molecules like AMPK and PGC-1α.
This is the first study of its kind to examine this particular diet and training strategy and its effects on performance outcomes in trained endurance athletes. Individual aspects of this protocol are supported by previous research, and the rationale for putting this strategy together appears to be very sound.
Frequently Asked Questions
This study used highly trained athletes. Could more recreational athletes or even people new to exercise expect to achieve similar effects?
This protocol would probably not be advisable for people that are new to exercise. The large majority of training adaptations will come from a more traditional approach to training, which would include replenishing muscle glycogen after hard workouts in order to be recovered for the subsequent training sessions. Recreational athletes who are more advanced in their training could find this beneficial, however.
Do the sessions have to be done three days in a row?
Maybe not. This is nearly impossible to answer without further research, but based on the mechanisms it seems that there would still be some benefit from doing this two to three times spread out across the week as long as there was a high intensity session in the afternoon or evening followed by a low-carb dinner and a low intensity endurance session undertaken after an overnight fast.
This study used men only, would the results be the same in women?
Possibly, but again this is very difficult to answer without more research. There is evidence that women may have more favorable adaptations from endurance training in the fed state (while men did better in the fasted state), however that particular study did not control for phase of the menstrual cycle. Testing for performance measures in women may also be complicated by the fact that endurance performance may be improved in the mid-luteal compared with early-follicular phases of the menstrual cycle.
What Should I Know?
Three weeks of training with strategic timing of carbohydrate intake (high-intensity training with high carbohydrate availability, recovery with low carbohydrate availability, and low intensity training with reduced muscle glycogen and in a fasted state) resulted in practical and meaningful performance improvements in trained triathletes, that were not seen in a control group that had the same total daily dietary intake and training protocol.
This study adds to the growing body of research showing that carbohydrate availability is an important mediator of the training response, and that strategies to maximize these adaptations can be effectively used.
Source: Enhanced Endurance Performance by Periodization of CHO Intake: “Sleep Low” Strategy